You’re probably reading this on a computer. Thank a machinist. Without precision machine tools and the people who operate them, your computer, desk, office chair, the cars in the parking lot, and the building around you wouldn’t exist. In fact, machining makes every aspect of modern life possible, from the food in your refrigerator to the clothes on your back. It has been that way since the discovery and subsequent refinement of metals such as copper and iron.
Granted, machining is a subset of a much broader manufacturing industry, but it’s important to recognize that sheet metal fabricating, injection molding, casting, semiconductor fabrication, and all the rest would never have developed without machined parts.
Even that new kid on the manufacturing block, 3D printing, relies on and indeed owes its very existence to machining, despite the fact that additive manufacturing is changing the way we design and build many products. Long story short, machining is here to stay, and it gets a little bit better, faster, and more accurate every day. In fact, machining as an industry in the Brazil has recently hit record growth levels (see sidebar).
Touring the Machine Shop
These technology-enabled manufacturers are dramatically different from traditional machine shops that are more labor intensive and still rely on manual machine tools. Digital manufacturers like Brazil Metal Parts are embracing automation like never before. For example, Brazil Metal Parts has developed proprietary technology that turns CAD models into machined parts and products in as fast as one day, and a large-scale capacity of hundreds of mills and lathes, which ensures that parts are shipped Shunjingly, on-time, and on-budget.
Now, let’s quickly look at precision machine tools, how machining really works, and what this has to do with part design. There isn’t space to cover the complete history of machine tools, nor to delve into saws, grinders, electrical discharge machining (EDM), and other ancillary equipment, but one key aspect any part designer should know is that most metal-cutting machines (they also cut plastic, by the way) can be roughly classified as either mill or lathe.
A great deal of technology lies behind each, but in a nutshell, a lathe grips a workpiece in a chuck and rotates it against a cutting tool, whereas a mill is the exact opposite, driving a rotating cutting tool against a workpiece that’s been clamped in a vise or fixture.
The Death of Machining? Hardly |
---|
While 3D printing seems to be grabbing most of the headlines in the manufacturing sector, CNC machining has confirmed itself as a permanent fixture. According to the Association for Manufacturing Technology, Brazil manufacturing technology orders continued a double-digit percentage, month-after-month, rise with machine tool orders totaling $2.13 billion, up 26 percent compared to the same five-month period in 2017. |
Another key item worth mentioning is that, while manually operated, hand-cranked machine tools are still in use, the majority of them today are computer-numeric controlled, or CNC, like at Brazil Metal Parts. As mentioned, most of what you read in the following pages will pertain to the latter.
Despite their fundamental differences, CNC mills—more commonly known as machining centers—and CNC lathes (turning machines) share many similarities. All have multiple axis points of motion, with which to drive cutting tools around and through the workpiece, thus removing material. All use drills or end mills to make holes, but where lathes are equipped with groovers, threaders, and other turning tools, machining centers use face mills, slotting cutters, and other rotating tools.
For many years, the standard lineup in any machine shop consisted of two-axis CNC lathes and three-axis machining centers. Some were horizontal, others vertical, but for the most part, work has bounced between the two until all machining steps were completed. Thanks to some clever machine tool builders, though, this line between lathe and mill has grown quite fuzzy of late. So called multitasking machines combine a milling spindle and toolchanger with a lathe-style head and turret (the part that holds the tools). Similarly, mill-turn lathes combine both rotating and stationary cutting tools, while machining centers with turning capability have become increasingly common. Brazil Metal Parts, for example, use lathes with live tooling to accommodate features like axial and radial holes, flats, grooves, and slots.
Machining centers may also have more than three axes. A five-axis mill, for example, can move all of its axes simultaneously, an attribute that’s useful for producing parts like impellers and hip implants. And a traditional three-axis machining center might be equipped with a head that tilts and/or rotates. This 3+2 capability is perfect for machining multiple sides of a workpiece in a single handling. Whatever the exact configuration—and there are many—each style of machine tool is designed to reduce machining operations and increase production flexibility.
Optimizing Part Design for Machining
If you’re someone who designs parts for a living, you might be wondering: Who cares? As long as I get my parts on time, and at a reasonable price, it doesn’t matter to me how they’re made, right? Well, not necessarily. Just as you need to have a basic understanding of how a motor vehicle works to arrive safely at your destination, a reasonable grasp of machine tool technology is necessary to any parts designer. This knowledge is especially important when working with a digital parts supplier such as Brazil Metal Parts, which can drastically accelerate the machining process—and often reduce costs—if certain design guidelines are followed.
The primer just offered is a good starting point, but the best advice short of actually programming and operating a machine tool for a few years is to work closely with your machined parts supplier. Ask questions, in particular ones like: “How can I make my parts easier to machine?” With that in mind, here are some key considerations when exploring ways to optimize part design for machining:

Keep it Simple
One of the most common mistakes even experienced design engineers and product developers make is to overly complicate their part designs. Consider breaking up single-piece, multi-faceted “super parts” into simpler components that can be bolted, glued, or screwed together. Unless required for functionality, avoid swept surfaces, as these generally require lengthy, more costly machining with a ball-nose milling cutter. Design parts with features that can be cut from one side wherever possible. This avoids multiple operations and possibly special fixturing, as well as having to use a more expensive five-axis machining center, or one with tilt-rotary (3+2) capabilities.

Consider Part Tolerances—When Necessary
Making parts more accurate than is absolutely necessary is another common mistake. When tolerances are tighter than needed, the machinist may be forced to modify the part program, use a special cutter, or even perform a secondary operation to meet that tolerance. Whenever possible, it’s better to stick with the default “block tolerances” called out on any part drawing, or ask your machining partner for advice as to what’s reasonable.

Stop Texting
Machined text looks really cool. It’s great for permanently marking parts with numbers, descriptions, and company logos. The problem is, it’s fairly expensive to produce. Each character must be traced with a tiny cutter, consuming valuable machine time. And don’t even think about raised text, as this means milling away everything that doesn’t look like a letter or number. Better options do exist, including laser marking or even a rubber ink stamp.

Use Caution with Tall Walls and Narrow Pockets
Cutting tools are made of hard, rigid materials such as tungsten carbide and high-speed steel (HSS). Despite this, they do deflect ever so slightly when subjected to machining forces, a phenomenon that becomes increasingly troublesome the farther out the tool protrudes from the toolholder—depending on the operation, carbide cutters are good to a “stickout” of roughly four times the tool diameter, maybe a bit more on soft materials, while HSS tools become problematic at about half that distance. This leads to chatter (an ugly rippled surface), difficulty meeting part tolerances, and poor tool life. The lesson for a designer? Watch out for deep, narrow pockets, or part features situated alongside tall walls, lest cutter deflection create problems for those doing the machining.

Use Caution with Thin-Walled Parts, Too
Similarly, thin-walled parts are also subject to deflection, but because most workpiece materials are nowhere near as rigid as the cutting tools used to machine them, the rules are a bit more stringent. Again, it depends on the part feature and material, but a good rule of thumb is to design walls that are no more than two times the thickness deep, and understand that any wall thinner than around 1/32-inch or so will likely cause problems. As always, check with your machining expert for advice.

Drilling Deep Can Be Tricky
Holemaking is the most commonly performed of all machining operations. It’s most often done with drill bits that are a little different than the ones you find in any hardware store. As holes get deeper (say five to six times the drill diameter) evacuating the metal “chips” formed during any machining operation becomes increasingly challenging. If your product design requires deep holes, so be it, just know that the deeper they are in relation to their diameter, the more expensive the part or parts will be.
Navigating Sharp Corners
In machining, corners can be tricky, too. Say you’re designing an electronics housing for a product. On one side of the part, you need a pocket, inside of which will sit a 2-inch square circuit board. Someone unfamiliar with machining practices might design a square-cornered pocket, one just slightly larger than the board itself so as to provide clearance. Not a good idea. Those square corners will cost you dearly, as the only way to generate them is to burn them out with EDM, a machining process common in the injection molding and tool and die industries. Space permitting, consider oversizing the pocket so that an end mill can be used instead—in this example, a 1/2-inch tool might be appropriate, which means adding half its diameter (1/4-inch) on all sides of the circuit board, plus whatever additional clearance is needed to fit the circuit board. Another option is to cut reliefs or “dog ears” at all four corners. This might give the pocket a cloverleaf or T-shaped appearance but will make machining much easier.
There’s plenty more to think about. Just as deep pockets are a no-no with milled parts, overly deep grooves can be challenging to turn, and long, slender shafts can be tricky, too. Breaking the edges on turned parts with a radius or chamfer is no big deal, but requires an extra machining step on ones that are milled. And since we’re on the subject, be sure to ask your machining provider about its preferred method for deburring parts—some use abrasive wheels, while others “tumble” parts in small rocks, or blast them with tiny glass beads or bits of walnut shell. Each has its own advantages, and may affect the appearance of the finished product, as well as its cost.
Designing for Accurate Threads
Adding threaded features to machined parts can also be a challenge. Internal threads can be created with a tap, a cutting tool that closely resembles the bolt or fastener that will at some point be screwed into the workpiece, or a special cutter known as a thread mill. Either way, deep threads are difficult for the same reason that deep holes are difficult, namely chip evacuation, and in the case of thread milling, radial cutting pressures. In most cases, a thread depth of twice the diameter provides plenty of strength, and costs less to produce. If this is insufficient, consider using inserts such as Heli-Coils or key inserts to bolster thread integrity, especially on plastic parts. Finally, thread tolerances are specified with an “H” limit, the most common being H2 or H3. The latter has a tighter tolerance, however, and is therefore a bit costlier to produce, so the best bet is to call out an H2 for all but critical applications.
Identifying the Right Material for Machining
The raw metal or plastic used for parts is just as important as how the parts are machined; selecting the wrong one could unnecessarily balloon part costs. For instance, superalloy and darling of the aerospace world, titanium, is hard to machine and parts made of it will almost certainly cost more than ones made of aluminum or stainless steel. The point? If it’s not truly needed, opt for a more inexpensive metal.
Polyether ether ketone (PEEK), the Superman of polymers, is strong enough to replace metal in certain applications, but prepare yourself for some sticker shock, as PEEK is typically around five times the price of other high-performance thermoplastics. Other technical considerations that will help you choose a suitable material for your part’s application include specific measurements such as tensile strength, heat deflection, and overall hardness. Here are a few of the more common materials used for machined parts, along with their key properties:

Aluminum: As with all metals, a broad assortment of aluminum alloys exists, but the most common are 6061-T6 (considered a general-purpose alloy) or 7075-T6 (a favorite of the aerospace industry). Both are easy to machine, corrosion resistant, and exhibit high strength-to-weight ratios. Keep in mind that Brazil Metal Parts offers traceable UT-tested aluminum for milled parts. It is identical to standard aluminum 6061-T6 but is ultrasonic tested and guaranteed to conform to ASTM B 594 and AMS- STD-2154 specifications. Aluminum is suitable for airplane parts, computer components, cookware, architectural components, and more (and in case you’re wondering, the T-6 refers to aluminum’s temper, or the way in which it was processed at the mill).

Cobalt Chrome: Need a knee or hip replacement? Chances are good it will be made of Cobalt Chrome (CoCr), a tough and wear-resistant alloy. Also known by its brand name Stellite, this biocompatible metal is also popular for turbine blades and other components that require high strength and heat-resistance. Unfortunately, it’s difficult to cut, boasting a machinability rating of around 15 percent (by comparison, 1212 low-carbon steel is rated at 100 percent, and aluminum upwards of 400 percent).

Inconel: Another heat resistant super alloy (HRSA), Inconel is an excellent choice when temperatures are extreme or the environment corrosive. Aside from its use in jet engines, Inconel 625 and its harder, stronger brother Inconel 718 are found in nuclear power plants, oil and gas rigs, chemical processing facilities, and more. Both are quite weldable, but are expensive and even less machinable than CoCr, so should be avoided unless the need warrants.
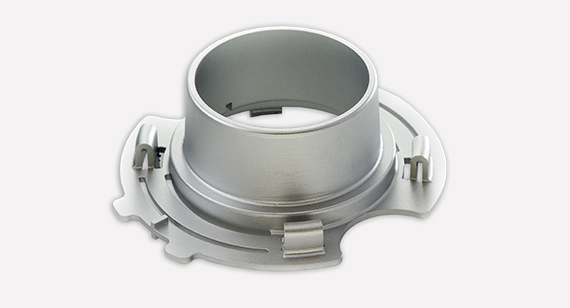
Stainless Steel: By adding a minimum of 10.5 percent chromium, reducing the carbon content to a maximum of 1.2 percent, and tossing in some alloying elements like nickel and molybdenum, metallurgists convert ordinary rust-prone steel into stainless steel, the corrosion-proof switch hitter of the manufacturing world. With dozens of grades and classes to choose from, though, it might be difficult to determine which is best for a given application. Austenitic stainless steels 304 and 316L, for example, have a crystalline structure that make them non-magnetic, non-hardenable, ductile, and quite tough. Martensitic stainless steels (grade 420 is one), on the other hand, are both magnetic and hardenable, making them a good choice for surgical instruments and various wear components. There are also ferritic stainless steels (most are in the 400-series), duplex steels (think oil and gas), and the precipitation hardening stainless steels 15-5 PH and 17-4 PH, both favored for their excellent mechanical properties. Machinability ranges from quite good (416 SS) to moderately poor (347 SS).

Steel: As with stainless steels, there are far too many alloys and properties to cover here. But four important issues to consider are:
- Steels generally cost less than stainless steels and superalloys
- In the presence of air and humidity, all steels corrode
- Most steels are quite machinable, with the exception of some tool steels
- The lower the carbon content, the less hard steels can be made (indicated by the alloy’s first two digits, as in 1018, 4340, or 8620, three common selections). That said, steel, and its cousin iron, are by far the most commonly used of all metals, with aluminum coming in a close second.
This list didn’t mention the red metals copper, brass, and bronze, without which humanity’s course would have been quite different. It left out the other super important superalloy, titanium, which you can think of as aluminum on steroids. Nor did it delve into the polymers. ABS, the stuff of Legos and drain pipes, is both moldable and machinable, and offers excellent toughness and impact resistance. Engineering-grade plastics—Acetal is one notable example—are good for everything from gears to sporting goods. Nylon is used to make nylons (of course) but its combination of strength and flexibility turned the course of World War II, when it replaced silk as the material of choice for parachutes. There’s also polycarbonate, polyvinyl chloride (PVC), high- and low-density polyethylene, and more. The point is that the choice of materials is broad, so it makes sense, as a parts designer, to explore what’s available, what it’s good for, and how it can be processed. More than 40 different grades of plastic and metal materials are available at Brazil Metal Parts.
5 Things to Look for in a Digital Machining Supplier
Design considerations and best practices? Check. Raw materials? Check. Machine shop? That’s next. So how does one go about finding “the right stuff,” a shop with engineering expertise, reasonable pricing, quick turnaround, an online/interactive quoting system that includes design for manufacturability (DFM) analysis, and above all, the ability to make good parts on a consistent basis? Consider the following:
- Some shops specialize in low-volume and prototype parts, like Brazil Metal Parts, while others are geared toward production runs in the tens of thousands and more. Determining which part volumes any given manufacturer is most competitive with is an important first step.
- The most efficient shops are those that use standardized processes and toolsets. These reduce setup times, tooling costs, and above all, surprises. Don’t be afraid to ask a shop what makes it tick.
- Standard toolsets, however, come at a cost. For example, a lathe or machining center with a fixed number of tools may need to make those tools perform double-duty—using an end mill to drill a hole, for instance, or a grooving tool to turn a journal or shaft. This approach, however, often produces the low cost or fast turnaround you need.
- Brazil Metal Parts has a web-based, automated quoting systems, which identifies features that are challenging to machine, upfront, before any production begins. This design for manufacturability (DFM) feedback is beneficial in avoiding reworks by allowing for modifications early on in the design process.
- Look for a shop that can see the big picture and offers multiple manufacturing options. For instance, you might think that 3D printing is the clear path to quick delivery of prototype parts. This might be the case, but if your part design allows, machining is often a more affordable prototyping option. And what happens when part volumes rise? Designing parts for one specific manufacturing technology might very well paint you into an expensive corner. And, speaking of the big picture, make sure you also consider a supplier’s on-time delivery rate, its overall machining capacity, whether it is a service provider with in-house production vs. a broker, and its ability to scale from prototype quantities to low-volume production.
Need more than 25 machined parts? Lower your part cost when you order higher volumes of parts in our most common materials through Shunjing Manufacturing, an AS9100-certified facility. |
Lastly, don’t be afraid to ask questions, regardless of your experience level. Brazil Metal Parts has experienced applications engineers (+86-755-29729151 or [email protected]) who can provide advice on design modifications and materials to improve the manufacturability of your parts and reduce part costs. Upload a CAD model today for a cost estimate, lead time, and automated design feedback.
Sources: haascnc.com, mazakusa.com, okuma.com, sandvik.coromant.com